Fallstudie A1
Case Study A1: Cluster Tox
Hybrid nanoparticles made up of polymeric and anorganic building blocks and larger clusters assembled thereof are key components in increasingly used and newly developed advanced materials.
Examples of hybrid nanomaterials
Example 1: The option of manipulating the light emitting properties in a dynamic manner makes polymer-coated nanoparticle ensembles to promising components in next-generation displays.1
Example 2: Hierarchically nanostructured anodes consisting of Silica nanoparticles in combination with poly(vinyl butyrale) / poly(acrylic acid) binding agent improve the performance of Lithium ion batteries.2
Example 3: The possibility to controll the decomposition of large nanopartilcle clusters linked by polymers into smaller nano objects for use in nanotheranostics.3
Physicochemical properties (e.g. dimension, shape, density of functional groups, surface heterogeneity, dispersity) of nanoparticles influence their interactions with molecules in biologic systems – and therewith their toxicity.
As for the effect those structural parameters of larger, hierarchical hybrid clusters on biological entities, very little is known at the moment. This motivates the research project A1: We want to resolve and understand the role of total size, number of molecules and macromolecular surface functionalisation of hybrid nanoclusters are affecting uptake by and distribution in cells, as well as their stability and toxicity.
Cluster preparation at IPF
At IPF, a modell system of so-called planet-satellite nanostructures will be synthesised.4 The syntesis can be done in a modular fashion, resulting in a library of structurally and compositionally different polymer-linked hybrid nanoparticle clusters.
The cluster systems will comprise of:
- distinct numbers and sizes of the constituent inorganic nanoparticles and,
- distinct polymer functionalization.
In particular, macromolecular nanoparticle linkers with varying amount of charged functional groups, with pH-responsive groups (mimicking weak polyelectrolytes employed in batteries) and pH-labile groups (mimicking the controlled decay of tumour-targeting clusters at their target site) will be employed.
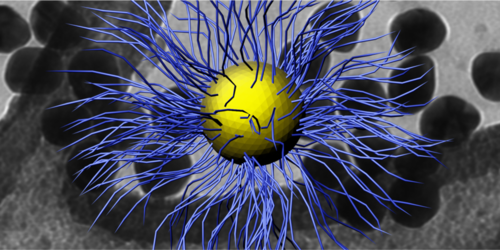
In-vitro analysis at INM
The cluster systems will be analysed in vitro in a lung model in terms of internalisation, subcellular localisation (confocal laser scanning and dark-field microscopy), lysosomal stability (dye accumulation, lysosomal protein release), and toxicity (cytotoxicity, ROS formation, cytokine release). All experiments involving clusters will be compared with the behaviour of the individual cluster components (i.e. the primary nanoparticles).
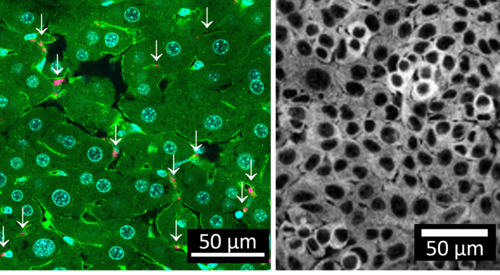
References and previous work
- Greybush NJ et al., Dynamic Plasmonic Pixels. ACS Nano 13:4 (2019) 3875. https://doi.org/10.1021/acsnano.9b00905
- Urbanski A et al., An Efficient Two-Polymer Binder for High-Performance Silicon Nanoparticle-Based Lithium-Ion Batteries: A Systematic Case Study with Commercial Polyacrylic Acid and Polyvinyl Butyral Polymers. J Electrochem Soc 166 (2019) A5275. http://dx.doi.org/10.1149/2.0371903jes
- Chou LYT, Zagorovsky K & Chan WCW, DNA assembly of nanoparticle superstructures for controlled biological delivery and elimination. Nat Nanotech 9 (2014) 148. https://doi.org/10.1038/nnano.2013.309
- Rossner C, Fery A, Planet-satellite nanostructures from inorganic nanoparticles: from synthesis to emerging application. MRS Communications 10 (2020) 112–122. https://doi.org/10.1557/mrc.2019.163
Involved Partners
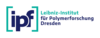
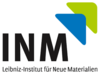